BY: JEFFREY G. SHAW
Jeffrey Shaw is genetics counselor, Cancer Center, Penrose—St. Francis
Health Services, Colorado Springs, CO.
The Third Pair of Articles in a Series about the Significance of Genetic
Science for Catholic Health Care
A Medical View
Approximately one in three Americans will develop a malignancy at some time
in his or her life. One in four deaths in the United States is due to cancer.
Cancer is second to heart disease as the most common cause of death in the nation.
Needless to say, cancer has an enormous impact on the lives of millions of people
and on the medical system that takes care of these people.
Cancer is caused by mutations in a variety of genes responsible for controlling
the growth of cells, either directly or indirectly. All cancer is "genetic"
in the sense that cancer is the uncontrolled division of a cell and the genes
control cell division. At the molecular level, therefore, all cancer is due
to mutation (a change in the genetic code) in our genes. These mutations are
usually caused by decades of exposure to carcinogens, which damage genes.
With the completion of the Human Genome Project (HGP), identification of genes
has progressed at an exponential rate, including identification of the genes
responsible for the control of cells. As a result of this new understanding
of the basic abnormalities that lead to cancer, the medical field called oncology
will drastically change over the next decade.
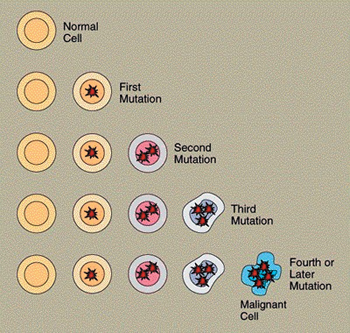
Cancer Basics
To understand how the genetic revolution will transform the treatment of cancer,
we need to review the basics of how cancer occurs. What is the difference between
normal cells and cancerous cells?
Normal cells:
- Reproduce in an organized, controlled, and orderly manner
- Do not divide when space or nutrients are inadequate
- Do not spread into parts of the body where they do not belong
- Become fully differentiated to perform specific tasks
- Have limited potential to replicate, over time lose their ability to do
so, and eventually die
Cancerous cells, on the other hand:
- Have uncontrolled cell growth, even when space and nutrients are lacking
- Have the ability to initiate new growth at distant sites
- Can become poorly differentiated, eventually looking different from the
cells where they originated
- Can escape detection and destruction by the immune system
We've said that most cancers are the result of genetic mutations caused
by exposure to "carcinogens." A carcinogen is any substance, situation,
or exposure that can damage genetic material (DNA). The hundreds of known carcinogens
include factors such as metabolic processes (e.g., free radicals, hormones),
viruses (e.g., hepatitis B, human papilloma virus), chemicals (e.g., tobacco,
alcohol, asbestos, heavy metals), and radiation (e.g., radiation therapy, ultraviolet
light, radioactive materials).
Genes and Cancer
Hundreds of genes have been identified as directly or indirectly participating
in a cell's ability to control growth. Given the success of the HGP, this
list of genes is sure to get longer. At present, genes controlling cell growth
are divided into four major categories: oncogenes, tumor suppressor genes, mismatch
repair genes, and "housekeeping" genes.
- Oncogenes result from an acquired mutation in normal genes called
"proto-oncogenes." The role of the proto-oncogenes is the signaling
of a cell to divide. Normal cells replicate to replace damaged or dying cells.
"Activation" describes the mutation in a proto-oncogene that transforms
it into an oncogene. These mutations push the cell to divide when it is not
supposed to. Therefore, the mutation of one proto-oncogene of a particular
pair (most genes occur in identical pairs, one from the mother and one from
the father) can lead to the initiation of cancer. Examples of oncogenes are
abl, myc, ras, and ret. (For a discussion of genetic "language,"
see Jeffrey G. Shaw, "An Introduction to Genetics," Health Progress,
May-June 2005, pp. 47-48.)
- Tumor suppressor genes actually have a variety of functions in the
control of a cell's growth. They are growth suppressing, playing an important
role in the regulation of cell growth, either directly or indirectly. One
can consider a tumor suppressor gene the opposite of an oncogene. If just
one copy of a particular tumor suppressor gene (either maternal or paternal)
is working in a cell, it will be sufficient to control cell growth. But loss
of function of both the maternal and paternal copy of the gene can lead to
unregulated cell growth. Examples of tumor suppressor genes are BRCA1, BRCA2,
APC, and WT1.
- Mismatch Repair Genes Every time a cell replicates and divides, all
three billion letters of genetic code must be duplicated perfectly to create
a new cell—a daunting task. Unfortunately, errors are made, and these
need to be corrected. Mismatch repair genes perform this function. They act
like "spell-checkers," automatically correcting mistakes. Damage
to both pairs of a mismatch repair gene will result in a loss of function,
and the cell will accordingly build up mutations every time it divides. Over
time, proto-oncogenes, tumor suppressor genes, and other genes involved in
cell growth can be damaged and cancer can occur. Examples of mismatch repair
genes are MLH1 and MSH2.
- Housekeeping Genes are difficult to summarize. There are hundreds
of housekeeping genes, and researchers are just beginning to identify their
roles. In general, housekeeping genes keep the cell clean and functional.
For example, housekeeping genes break down carcinogens that enter a cell,
regulate estrogen in the cell, and protect against viral activation of cancer
in the cervix. These genes generally do not participate directly in cell growth
regulation. Instead, their function seems to be directed toward protection
of the cell from carcinogenic invaders or processes.
Cancer Risks
Everyone has a "general population risk"—a risk level based
on the occurrence of cancer in a given population—to develop a type of
cancer. The risk level can be increased or decreased, depending on a person's
environmental exposures and lifestyle. For example, a person who uses tobacco
products increases his or her risk level for lung, throat, colon, and many other
types of cancer over the risk level of the general population. People with significant
exposure to ultraviolet light (from sunlight or tanning beds, for example) have
an increased risk for skin cancers.
Given the complexity of environments, reproductive decisions, physical development,
occupations, and differing lifestyles, it is unlikely that any one person would
have a general population risk for all types of cancer. A person's risk
for a specific type of cancer can be determined as falling into one of three
general categories.
Sporadic Most cancer occurs in a sporadic pattern. Because the patient
has no family history for it, the disease appears to have "come out of
nowhere." In such cases, the tumor suppressor, mismatch repair, and other
important genes inherited from parents are fully functional at birth. The cancer
is caused primarily by multiple exposures to carcinogens. Sporadic cancers—breast,
colon, prostate, and ovarian cancer are examples—tend to occur later in
life, usually after age 50. By that time, the person is likely to have accumulated
many mutations, and his or her immune system is likely to be less proficient
in protecting against cancer cells. However, sporadic cancers also occur in
childhood or youth (i.e., testicular cancer).
Inherited In cases involving an inherited (sometimes called a "high
penetrance") cancer predisposition, the person has inherited a faulty tumor
suppressor, oncogene, or mismatch repair gene from a parent. Because the mutated
(nonfunctional) gene was present in the egg or sperm, it is present in every
cell of the body. Some sort of environmental insult will be necessary to mutate
the other gene of the pair sufficiently to initiate the possibility of cancer.
But because of the inherited mutation, the person will have a significant increase
in risk for malignancy, usually in specific organs.
The cancer in such cases often has an early onset, and the risk for second
primary tumors is increased significantly. Inherited cancer predispositions
do not "dilute." Either a child inherits the faulty gene from a parent
and has a significantly increased risk for cancer, or the child does not inherit
the faulty gene and does not have an increased risk (depending on the family
history of cancer).
For example, people with an inherited predisposition, because of an inherited
mutation in either the BRCA1 or BRCA2 tumor suppressor genes, will have a 44
to 85 percent lifetime risk of developing the disease, instead of a normal risk
of about 10 percent. And people with a high risk will also have a 50 percent
chance of developing the disease before age 50, instead of the usual 2 percent
risk. Their risk for developing a brand new breast cancer will run as high as
60 percent, as will their risk for a primary ovarian cancer.
Familial In familial (sometimes called "multifactorial low penetrance")
cancer predispositions, the person involved has inherited several housekeeping
genes that, although functional, are not doing a good job of protecting the
patient from carcinogens. Affected families usually have an excess of cancer
cases, but the illness does not necessarily occur in youth. The cancer toward
which the family is predisposed need not be genetically related. (For instance,
the tumor suppressor genes controlling the growth of cervical cells differ from
those controlling breast cells; breast and cervical cancer, for example, are
not genetically related).
Familial predispositions are "multifactorial" conditions. In other
words, the patient involved must inherit several suboptimal housekeeping genes
and be exposed to specific carcinogens. As a result, familial predispositions
tend to "dilute" over each generation because it is difficult to pass
down several specific genes, and families are usually not exposed to the same
environmental conditions over several generations. Therefore, familial predispositions
tend to confer a small to moderate increase in the risk for cancer.
Impact on Medical Care
We are today experiencing an explosive growth of knowledge regarding basic
alterations of cells that lead to cancer. This knowledge has begun a process
that will vastly change the medical management of cancer patients.
Diagnosis/Prognosis The specific characterization of the genetic damage
that has occurred in a tumor will provide physicians with a more clearly defined
understanding of the tumor's aggressiveness, as well as help them predict
risk for metastasis (spread of a cancer to other parts of the body) and survival.
Testing breast tumors for overexpression of the gene Her2-neu is now commonplace.
Women with increased expression of this gene are known to have more aggressive
cancers. Identification of this abnormality has led to development of a new
drug called Herceptin, which blocks the Her2-neu protein and improves the patient's
chances for survival.
New tumor multigene analysis is today helping stage I breast cancer patients
determine whether their risk of recurrence is high or low. For example, a woman
might be diagnosed (on the basis of tumor size, lymph node involvement, and
other signs) as having an early-stage breast cancer, which would ordinarily
indicate a relatively low chance of recurrence. Suppose, however, that multigene
analysis shows a genetic "footprint" of the tumor indicating a much
higher chance of recurrence. The woman could then opt for more aggressive management
of her initial tumor, thereby reducing the risk of recurrence. By the same token,
multigene analysis showing a low chance of recurrence could help prevent overtreatment.
Recent research performed by Mingxin Che, MD, PhD, at Wayne State University,
Detroit, is likely to help patients with prostate cancer.1 Che and
his colleagues evaluated the expression of the P53 oncogene in the tumors of
prostate patients. P53 is a well-known oncogene that, when activated, promotes
tumor growth. Studies done by Che and his colleagues showed that men having
prostate tumors with abnormally high levels of the P53 protein were twice as
likely to develop distant metastases at five years and had a higher than normal
mortality rate.
Pharmacogenetics The tailoring of drugs for patients whose individual
response can be predicted by gene expression profiles, or "fingerprinting"
of the tumor, can help identify those likely to benefit from a specific treatment
and those not likely to benefit. For example, "fingerprinting" a tumor
can indicate which form of chemotherapy is likely to produce the fewest side
effects in the patient and improve his or her prognosis.
Tumor "fingerprinting" of this kind has been helpful in showing why
standard therapies for acute lymphoblastic leukemia cure 80 percent of children
afflicted by the disease, even though the same drug therapy fails the other
20 percent. Research done late last year by Pier Paolo Pandolfi, MD, PhD, indicated
that young patients with a working PTEN gene in their tumors are more responsive
than others to the drug Herceptin.2
About 10 percent of patients with chemotherapy-resistant colon cancer respond
to two different monoclonal antibodies. These drugs target the epidermal growth
factor receptor (EGFR). Current studies show that testing of the EFGR gene can
help identify people who will benefit from these therapies. By the same token,
the test also identifies those who, because they won't benefit from the
medication, should not be exposed it.
Therapy and Risk Prediction
Currently, most cancer therapeutics operate according to a single paradigm:
They damage DNA in rapidly dividing cancer cells, thereby killing those cells.
In the case of chemotherapeutic treatments, this is not an ideal way to target
a cell, because many noncancerous cells of the body also divide rapidly—for
example, hair cells and the cells lining the gastrointestinal tract. As medical
science increases its genetic understanding of the ways cancer occurs, it will
develop new ways to attack a tumor. Comprehension of a tumor's genetic
nature has already led to the development of Gleevec, for gastrointestinal tumors
and one type of leukemia, and Herceptin, for some types of breast cancer. Targeted
therapies will be developed (and improved) for other kinds of cancer.
As for predicting cancer risks, presymptomatic genetic testing is currently
available for a number of inherited cancer predispositions. In families with
histories suggesting an inherited predisposition, such testing can show which
family members have a high risk for developing cancer and which do not. No special
intervention would be necessary for those determined not to have an increased
risk. For those found to have an increased risk, surgical or chemotherapeutic
intervention can drastically reduce the risk for cancer. Furthermore, screening
techniques for people at risk can be altered to help detect a possible cancer
at the earliest stage, when it will be most amenable to treatment.
However, highly penetrant, inherited cancer predispositions account for only
a small proportion of all cancer occurrences. Medical science must expand testing
to cover the more common, lower-penetrated cancer predispositions. A recent
study indicates that people with a common specific variant in the CASP8 gene
(a gene involved with programmed cell death) have an approximately 40 percent
lower risk of developing breast cancer than people with a different variant.3
Given improved understanding of the gene variants that protect us from cancer,
we will be able to test greater numbers of people to determine whether they
are at an increased or decreased risk for cancer.
Oncology and the Future
Knowledge about the fundamental changes in cell genes that lead to cancer is
sure to greatly affect risk assessment, diagnosis, and treatment. These advances
will significantly alter the practice of medical oncology. Identification of
people with inherited or familial risks for cancer will show us those who are
likely to benefit from preventive interventions, including screening to identify
tumors at an early stage.
Advances in genomic technology will improve our ability to predict when a tumor
is likely to metastasize to other parts of the body—and when the patient
might, accordingly, benefit from a more aggressive therapy. It will aid us in
understanding which types of treatments will be beneficial to a specific patient
and which will not.
New biologic therapeutic treatments for cancer promise to be more effective—and
to have fewer toxic side effects—than currently available treatments. The
biggest challenge will likely be incorporating this wealth of new information
into clinical practice.
NOTES
- M. Che, M. DeSilvio, A. Pollack, et al., "Prognostic Value of Abnormal
P53 Expression in Locally Advanced Prostate Cancer Treated with Androgen Deprivation
and Radiotherapy: A Study Based on RTOG 9202" (paper presented at the
American Society of Clinical Oncology's 2005 Prostate Cancer Symposium, Orlando,
FL, February 19, 2005); abstract at www.asco.org/ac/1,1003_12-002643-00_8-0037-00_19-0020372,00.asp.
- P. P. Pandolfi, "Breast Cancer—Loss of PTEN Often Predicts Resistance
to Treatment," New England Journal of Medicine, November 25, 2004,
pp. 2,337-2,338.
- G. MacPherson, C. S. Healey, M. D. Teare, et al., "Association of a
Common Variant of the CASP8 Gene with a Reduced Risk of Breast Cancer,"
Journal of the National Cancer Institute, vol. 96, no. 24, December
15, 2004, pp. 1,866-1,869.
Copyright © 2005 by the Catholic Health Association of the United States
For reprint permission, contact Betty Crosby or call (314) 253-3477.